Operating system virtualization/partitioning
The operating system virtualization technique allows the same physical host to serve different workloads and isolate each of the workloads. Please note that these workloads operate independently on the same OS. This allows a physical server to run multiple isolated operating system instances, called containers. There is nothing wrong if we call it container-based virtualization. The advantage of this type of virtualization is that the host operating system does not need to emulate system call interfaces for operating systems that differ from it. Since the mentioned interfaces are not present, alternative operating systems cannot be virtualized or accommodated in this type of virtualization. This is a common and well-understood limitation of this type of virtualization. Solaris containers, FreeBSD jails, and Parallel's OpenVZ fall into this category of virtualization. While using this approach, all of the workloads run on a single system. The process isolation and resource management is provided by the kernel. Even though all the virtual machines/containers are running under the same kernel, they have their own file system, processes, memory, devices, and so on. From another angle, a mixture of Windows, Unix, and Linux workloads on the same physical host are not a part of this type of virtualization. The limitations of this technology are outweighed by the benefits to performance and efficiency, because one operating system is supporting all the virtual environments. Furthermore, switching from one partition to another is very fast.
Before we discuss virtualization further and dive into the next type of virtualization, (hypervisor-based/software virtualization) it would be useful to be aware of some jargon in computer science. That being said, let's start with something called "protection rings". In computer science, various hierarchical protection domains/privileged rings exist. These are the mechanisms that protect data or faults based on the security enforced when accessing the resources in a computer system. These protection domains contribute to the security of a computer system.
As shown in the preceding figure, the protection rings are numbered from the most privileged to the least privileged. Ring 0 is the level with the most privileges and it interacts directly with physical hardware, such as the CPU and memory. The resources, such as memory, I/O ports, and CPU instructions are protected via these privileged rings. Ring 1 and 2 are mostly unused. Most of the general purpose systems use only two rings, even if the hardware they run on provides more CPU modes (https://en.m.wikipedia.org/wiki/CPU_modes) than that. The main two CPU modes are the kernel mode and user mode. From an operating system's point of view, Ring 0 is called the kernel mode/supervisor mode and Ring 3 is the user mode. As you assumed, applications run in Ring 3.
Operating systems, such as Linux and Windows use supervisor/kernel and user mode. A user mode can do almost nothing to the outside world without calling on the kernel or without its help, due to its restricted access to memory, CPU, and I/O ports. The kernels can run in privileged mode, which means that they can run on ring 0. To perform specialized functions, the user mode code (all the applications run in ring 3) must perform a system call (https://en.m.wikipedia.org/wiki/System_call) to the supervisor mode or even to the kernel space, where a trusted code of the operating system will perform the needed task and return the execution back to the user space. In short, the operating system runs in ring 0 in a normal environment. It needs the most privileged level to do resource management and provide access to the hardware. The following image explains this:
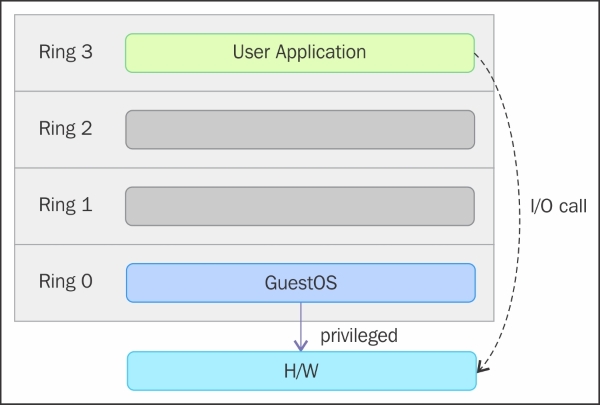
The rings above 0 run instructions in a processor mode called unprotected. The hypervisor/Virtual Machine Monitor (VMM) needs to access the memory, CPU, and I/O devices of the host. Since, only the code running in ring 0 is allowed to perform these operations, it needs to run in the most privileged ring, which is Ring 0, and has to be placed next to the kernel. Without specific hardware virtualization support, the hypervisor or VMM runs in ring 0; this basically blocks the virtual machine's operating system in ring-0. So the VM's operating system has to reside in Ring 1. An operating system installed in a VM is also expected to access all the resources as it's unaware of the virtualization layer; to achieve this, it has to run in Ring 0 similar to the VMM. Due to the fact that only one kernel can run in Ring 0 at a time, the guest operating systems have to run in another ring with fewer privileges or have to be modified to run in user mode.
This has resulted in the introduction of a couple of virtualization methods called full virtualization and paravirtualization, which we will discuss in the following sections.
Full virtualization
In full virtualization, privileged instructions are emulated to overcome the limitations arising from the guest operating system running in ring 1 and VMM runnning in Ring 0. Full virtualization was implemented in first-generation x86 VMMs. It relies on techniques, such as binary translation (https://en.wikipedia.org/wiki/Binary_translation) to trap and virtualize the execution of certain sensitive and non-virtualizable instructions. This being said, in binary translation, some system calls are interpreted and dynamically rewritten. Following diagram depicts how Guest OS access the host computer hardware through Ring 1 for privileged instructions and how un-privileged instructions are executed without the involvement of Ring 1:
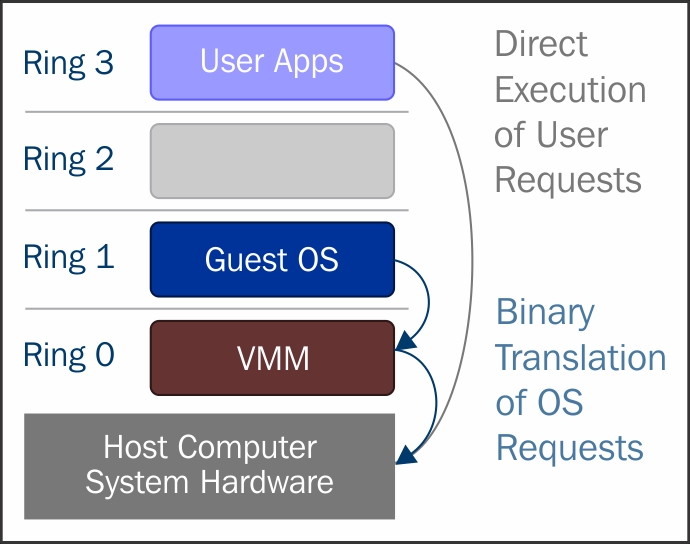
With this approach, the critical instructions are discovered (statically or dynamically at runtime) and replaced with traps into the VMM that are to be emulated in software. A binary translation can incur a large performance overhead in comparison to a virtual machine running on natively virtualized architectures.
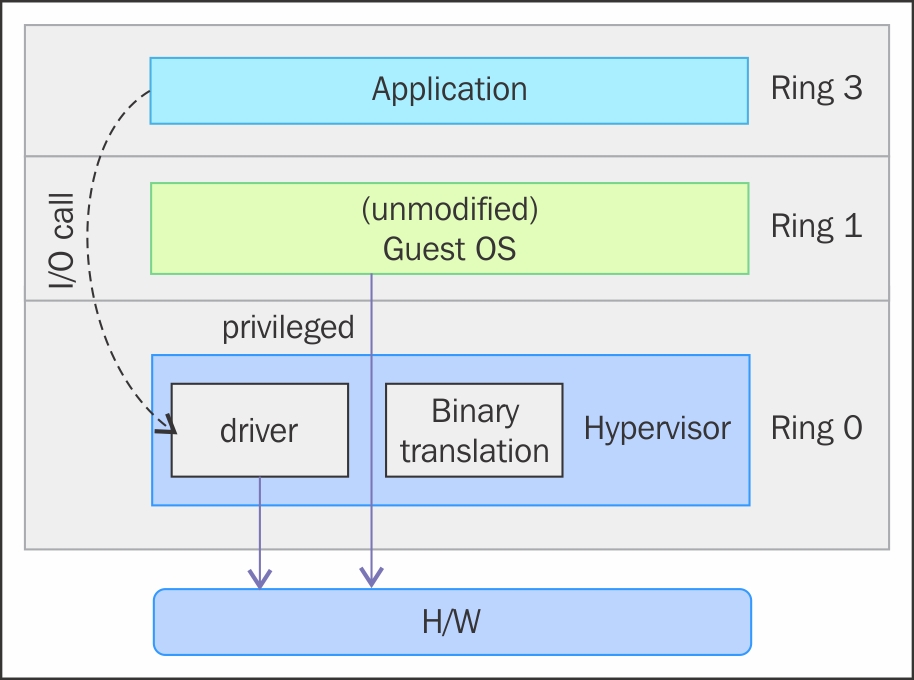
However, as shown in the preceding image, when we use full virtualization we can use the unmodified guest operating systems. This means that we don't have to alter the guest kernel to run on a VMM. When the guest kernel executes privileged operations, the VMM provides the CPU emulation to handle and modify the protected CPU operations, but as mentioned earlier, this causes performance overhead compared to the other mode of virtualization, called paravirtualization.
Paravirtualization
In paravirtualization, the guest operating system needs to be modified in order to allow those instructions to access Ring 0. In other words, the operating system needs to be modified to communicate between the VMM/hypervisor and the guest through the "backend" (hypercalls) path.
Note
Please note that we can also call VMM a hypervisor.
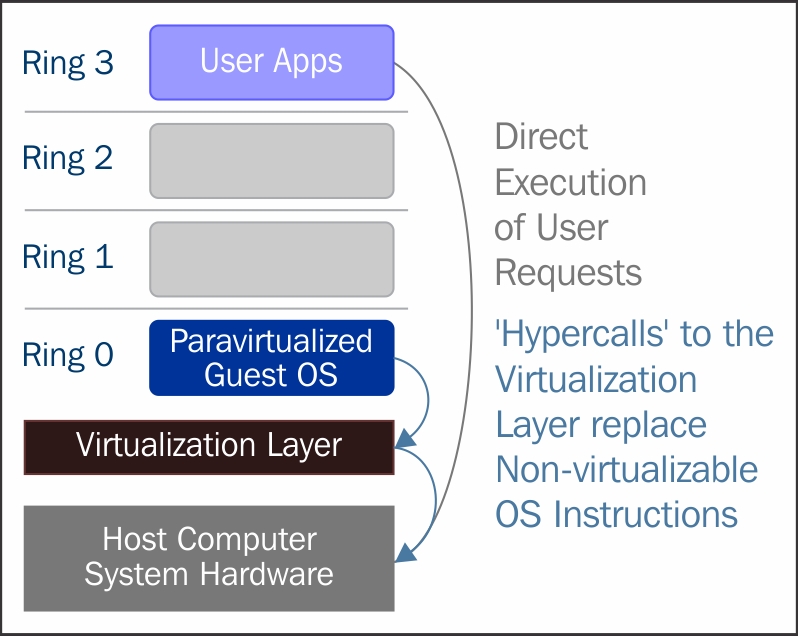
Paravirtualization (https://en.wikipedia.org/wiki/Paravirtualization) is a technique in which the hypervisor provides an API and the OS of the guest virtual machine calls that API which require host operating system modifications. Privileged instruction calls are exchanged with the API functions provided by the VMM. In this case, the modified guest operating system can run in ring 0.
As you can see, under this technique the guest kernel is modified to run on the VMM. In other terms, the guest kernel knows that it's been virtualized. The privileged instructions/operations that are supposed to run in ring 0 have been replaced with calls known as hypercalls, which talk to the VMM. The hypercalls invoke the VMM to perform the task on behalf of the guest kernel. As the guest kernel has the ability to communicate directly with the VMM via hypercalls, this technique results in greater performance compared to full virtualization. However, This requires specialized guest kernel which is aware of para virtualization technique and come with needed software support.